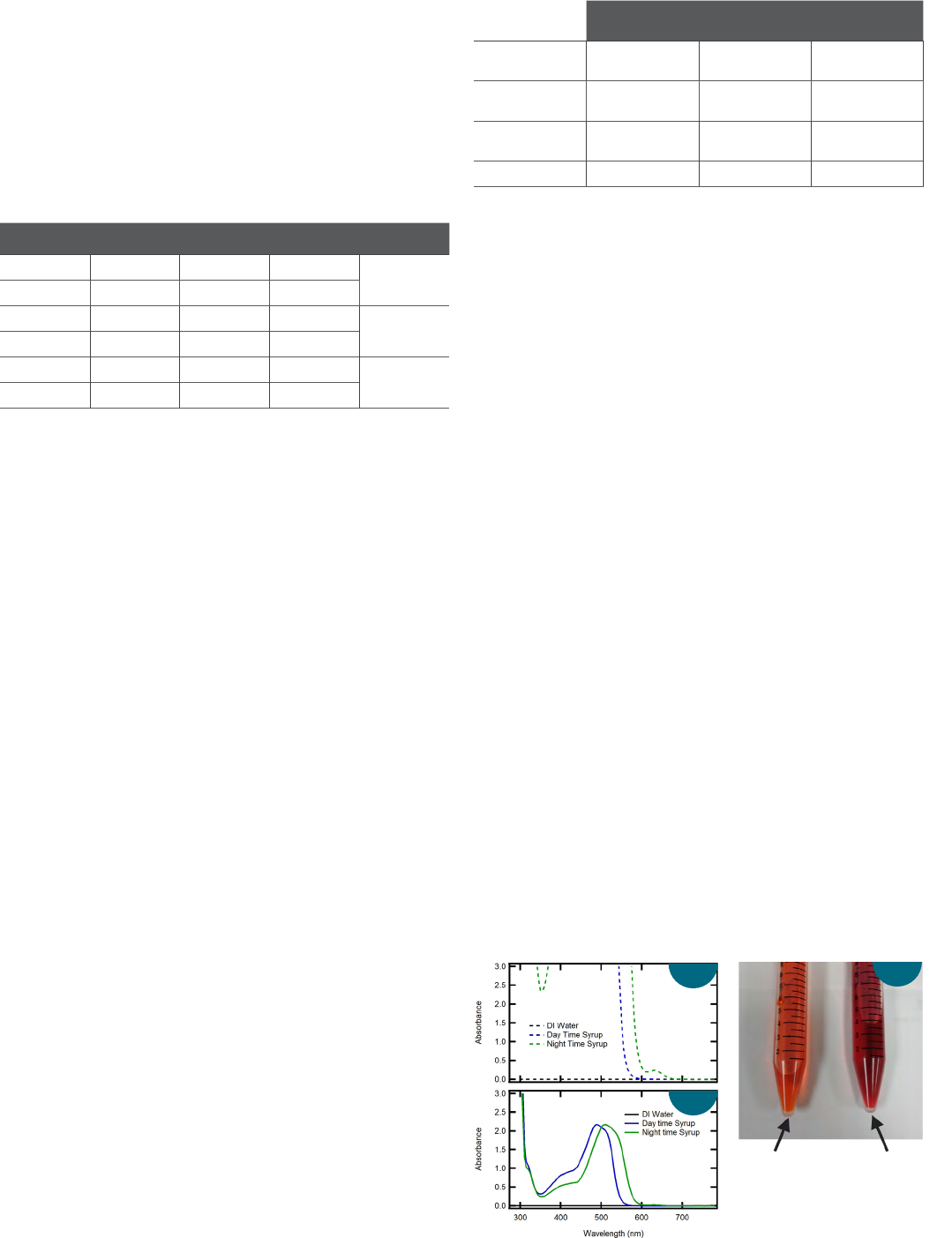
From these spectra, it is clear there are small differences in the
transmittance, and consequently absorption, of each matching
solution; however, color difference calculations were needed to
rigorously compare the colors. As described previously, the CIE
L*a*b* values were calculated using the Insight Pro Software.
A select set of color-matching standards were chosen for
comparison and are included in Table 2 as these standards
(Soln. A and B, Soln. J and K, and Soln. Q and R) appear similar
enough to each other in color that they are difficult to tell apart.
Table 2: CIE Lab and color difference values for select USP color matching
solutions (A, B, J, K, Q, R). Color difference calculations were carried out
for samples which appear similar by eye.
Solution L* a* b* ΔE*
A 87. 5 0.5 28.5
9.7
B 83.3 2.4 37. 0
J 69.1 12.0 80.0
12.5
K 73.9 12.5 91.5
Q 85.1 2.6 28.3
5.2
R 88.1 2.5 24.0
The color difference values calculated between matching
solutions A and B, J and K, and Q and R are relatively low;
however, a numerical limit is required to put these difference
values into context. In the pharmaceutical industry, different
formulations may require different methods of comparison
against a color-matching standard. For example, one product
may need to have no discernable color (achromatic), while
another must meet a minimum color value. Consequently,
USP has developed a set of criteria which can be used to set
acceptable limits for the calculated color difference from a
standard (Table 3).
There are four main test limits which can be used depending
on the color expectations for the analyzed product. Each test
defines a limit to an acceptable color difference between the
material and a given standard. For a sample which should have
no color, the first test in Table 3 (colorless/achromatic) defines
the necessary color difference limit as ΔE* < 1, where the color-
matching standard is purified water.
For samples where the sample has an expected color, there
are a few different options for analysis. If the color must
match a given standard color exactly, the second test in
Table 3 (Indiscernible from Standard) is required. Here, the
color difference between the product and the color matching
standard is used and must be less than 3. As mentioned
previously, this defines the color difference that is discernable
by the human eye.
10
The last two analyses define maximum
and minimum color limits. Here, a sample can either be more
or less colorful than a given standard. USP defines Δh
ab
*, the
difference in hue between the sample and matching standard
chosen must be less than 15. When setting the maximum or
minimum color limit, instead of comparing the color difference
against a number, two different analyses are required: one
where the color of the standard is compared to the color of
pure water (ΔE
std
*) and one where the color of the product is
compared against pure water (ΔE*).
As the color difference values shown in Table 2 are intended
to determine how similar the color of the two solutions are to
one another, this analysis would follow the “Indiscernible from
Standard” test. The passing criteria would require a calculated
color difference of less than 3. For each set of standards, the
color difference exceeds this limit, indicating they fail this test
and are distinguishable from one another. This result highlights
how small differences in color can be analyzed through the
instrumental method, where it is difficult to perceive visually.
Analysis of liquid samples—cough syrup
The color-matching standards are ideal solutions with
optimized component concentrations to produce a measurable
spectrum in a standard 10 mm cuvette. Real samples may not
be manufactured to produce UV-Visible absorption spectra that
can be easily measured under these conditions. For example,
Figure 4a includes the absorption spectra of a “Daytime” and
“Night-time” cough syrup measured in a 10 mm cuvette. By
eye, the “Daytime” syrup appears orange while the “Night-time”
syrup appears red/purple.
As shown, both samples absorb greatly at wavelengths shorter
than 550 nm (A > 3). In UV-Visible absorption measurements,
it is good practice not to use highly absorptive samples for
calculations or quantification, as very little light is allowed to
pass through the sample and be detected by the system. For
example, an absorption of 3 indicates 99.9% of the incident
light is absorbed by the sample, leaving 0.1% of the light
collected by the detector. Consequently, the absorption spectra
in Figure 4a are not ideal for color analysis and result in the
values described in Table 4.
Figure 4: Absorption spectra of "Daytime" and "Night-time" cough syrup
collected using a (a) 10 mm and (b) 1 mm quartz cuvette. (c) An image of the
"Daytime" and "Night-time" cought syrup).
Daytime Night-time
a
b
c
Table 3: Passing criteria for color difference tests from USP <631>.
10
For the
maximum and minimum color difference measurements, ΔE
std
* refers to the
color difference between a matching standard and purified water while ΔE*
refers to the color difference of the sample against purified water.
Test
Color
Standard
Passing
Criteria
1 Colorless
(Achromatic)
Purified Water ΔE* < 1
2 Indiscernible
from Standard
Color Matching
Solution
ΔE* < 3
3 Maximum
Color
Purified Water ΔE* < ΔE
std
*
4 Minimum Color Purified Water ΔE* > ΔE
std
*